Neuroscience
How Brain Cells Communicate to Allow Humans to Adapt
To adapt, two brain cells must communicate effectively. This is how they do it.
Posted April 30, 2021 Reviewed by Ekua Hagan
Key points
- Brain adaptation depends on the needs of neural networks being communicated to the cells maintaining synchrony.
- Oligodendrocyte precursor cells form intimate connections with nerve fibers to respond to increased neural activity.
- Oligodendrocytes monitor neural activity and change brain structure to speed up or slow down neural signals.
- Astrocytes act as an intermediate communicator between neurons and oligodendrocytes.
Adaptability may be the defining characteristic of our species — not our large brains, our long legs, or our ability to make tools, but our ability to rapidly learn new skills and behaviours in quickly changing environments. Adaptability has enabled us to thrive and inhabit just about every environment on the planet since we left Africa just 60,000 years ago.
Just one example of our immense capacity for adaptation is found in the tissue of the brain and spinal cord—collectively known as the central nervous system, or CNS. Born of a relationship formed between two types of cells millions of years ago, the dynamic duo of the CNS (axons and myelin) rapidly adapts to meet the demands imposed upon it.
This is the third part of the dynamic duo series. In part 1, we talked about how the axon-myelin relationship allows for increased brain processing power. In part 2, we talked about how myelin coordinates neural networks. In part 3, we’re going to cover how myelin adapts to the needs of neural networks: how the subtle tweaks in conduction velocity needed are communicated from the axon to the oligodendrocyte. This is a process called adaptive myelination and it represents a form of plasticity within the CNS.
What follows are three proposed mechanisms of adaptive myelination, each with varying levels of experimental support. As research in the field progresses, increased support for one mechanism does not necessarily negate the role of other mechanisms. As with all things in biology, it’s messy. It’s likely all mechanisms function to support adaptive myelin changes to some degree. And the mechanism that dominates may change depending on brain conditions and context.
Oligodendrocyte Precursor Cells
In the CNS, myelin is produced by oligodendrocytes. These octopus-like looking cells nestle among tracts of axons, their arms extending away from their central body to concentrically wrap sections of long, wiry nerve fibers.
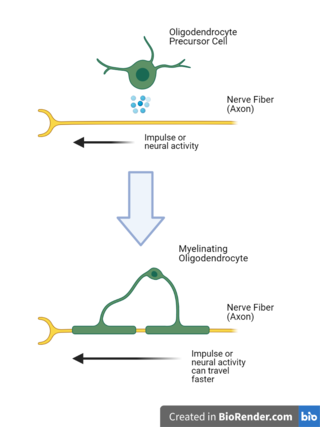
Oligodendrocytes develop from oligodendrocyte precursor cells, also known as OPCs. OPCs are all over the CNS and, much like their differentiated successors (oligodendrocytes), are in close proximity to axons.
OPCs form intimate connections with axons called synapses. A synapse is a junction where electrical signals from neurons are communicated to another cell. The neuron-neuron synapse is the mostly widely known and was the first to be discovered; however, neurons form synapses with other brain cells too. The synapse between the axon and OPC is one such example.
The axon-OPC synapse is mediated by a neurotransmitter called glutamate. As the electrical impulse travels along the axon, calcium ions trigger the release of glutamate into the space between the axon and OPC. Receptors on the OPC bind the neurotransmitter, promoting the survival of the cell and the production of proteins necessary for the formation of new myelin.
New myelin on a previously bare axon allows electrical impulses to travel faster. By responding to neural activity and developing into oligodendrocytes that produce myelin, OPCs enable activated and highly active portions of a neural network to increase their conduction speed.
Oligodendrocytes
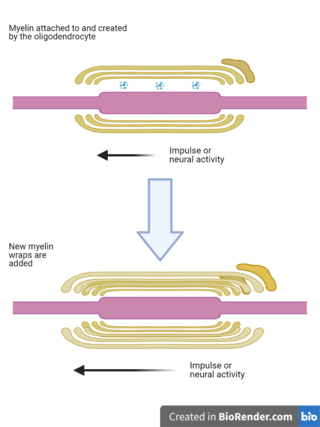
For some oligodendrocytes, the axon-OPC synapse persists as the cell matures and produces myelin. At this stage, the synaptic machinery becomes restricted to the layer of myelin closest to the axon.
This synapse—now called the axo-myelinic synapse—places myelin itself in a prime location to monitor and respond to the needs of neural networks.
Neural activity will trigger the release of glutamate from their axons, which will bind to receptors on the inner surface of myelin causing calcium fluctuations within myelin.
Calcium sensitive proteins residing within myelin can then promote additional myelin wraps to speed up electrical impulses. Or, impulses could be slowed by the triggering of proteins capable of degrading proteins holding concentric wraps together.
Astrocytes
Another type of glia (non-neuronal cell), called astrocytes, play a substantial role in helping the CNS dynamic duo adapt to the fluctuating needs of neural networks.
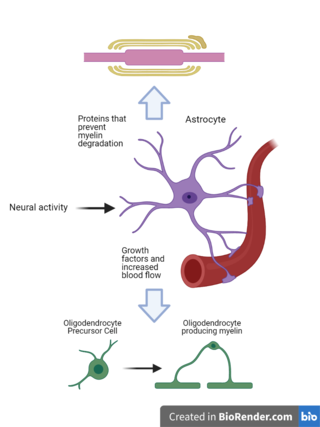
More star shaped than oligodendrocytes with processes more fibrous than OPCs, astrocytes are in contact with all parts of the neuron. Furthermore, astrocytes are close enough to OPCs, oligodendrocytes and myelin that their activity has immediate effects on these cells and structures.
Neuronal activity influences the proteins an astrocyte creates. Some of these proteins may be ones known to stimulate the development of OPCs into oligodendrocytes—like PDGF, FGF, and LIF.
These cells also remove energetic barriers to myelin generation by increasing blood flow to active brain regions.
Perhaps the most direct roll the astrocyte plays in adaptive myelination is through the production and secretion of proteins that prevent myelin degradation.
As a stake is used to anchor a tent to the ground so it doesn’t easily blow away, each layer of myelin is anchored to the axon by a protein. Degradatory proteins called proteases can sever these protein anchors, stripping away layers of myelin.
Astrocytes produce neutralizing proteins that prevent these proteases from degrading myelin. And astrocytes produce them in response to neural activity.
It’s the perfect mechanism for fine tuning myelin. If the neuron is inactive—because the portion of the neural network simply isn’t used, or because it isn’t properly synced—the astrocyte will not be stimulated to produce the neutralizing protein and proteases will degrade myelin. The speed of electrical impulses will slow as a result.
An active neuron will produce the opposite effect: The astrocyte will be stimulated to produce the neutralizing protein, preventing myelin degradation and preserving its integrity. Electrical impulse speed is maintained.
References
Bouhrara M, Alisch JSR, Khattar N, Kim RW, Rejimon AC, Cortina LE, Qian W, Ferrucci L, Resnick SM, Spencer RG (2020) Association of cerebral blood flow with myelin content in cognitively unimpaired adults. BMJ Neurol Open 2:e000053.
Dutta DJ, Woo DH, Lee PR, Pajevic S, Bukalo O, Huffman WC, Wake H, Basser PJ, SheikhBahaei S, Lazarevic V, Smith JC, Fields RD (2018) Regulation of myelin structure and conduction velocity by perinodal astrocytes. Proc Natl Acad Sci 115:11832–11837.
Hasel P et al. (2017) Neurons and neuronal activity control gene expression in astrocytes to regulate their development and metabolism. Nat Commun 8:15132.
Lehmann N, Villringer A, Taubert M (2020) Colocalized White Matter Plasticity and Increased Cerebral Blood Flow Mediate the Beneficial Effect of Cardiovascular Exercise on Long-Term Motor Learning. J Neurosci 40:2416–2429.
MacVicar BA, Newman EA (2015) Astrocyte regulation of blood flow in the brain. Cold Spring Harb Perspect Biol 7.
Micu I, Plemel JR, Caprariello AV, Nave K-A, Stys PK (2018) Axo-myelinic neurotransmission: a novel mode of cell signalling in the central nervous system. Nat Rev Neurosci 19:49–58.
Stadelmann C, Timmler S, Barrantes-Freer A, Simons M (2019) Myelin in the Central Nervous System: Structure, Function, and Pathology. Physiol Rev 99:1381–1431.